Magnetic Impulse Torque Converter Project
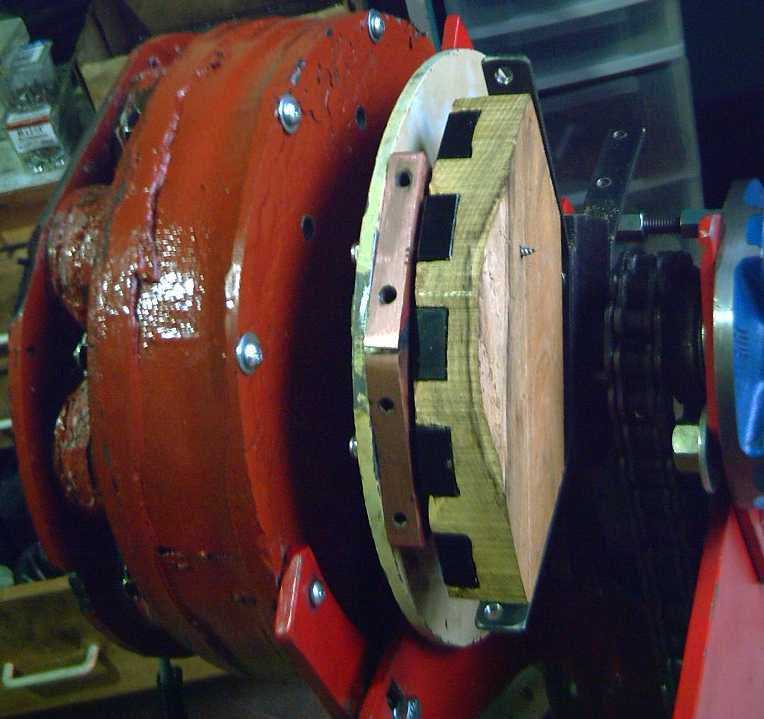
Experimental Version 2: Copper wedge on motor rotor,
and 'wedge' of 5 supermagnets on output "rotor".
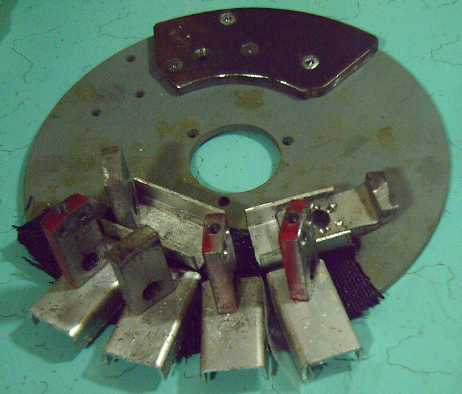
Gluing PP strapping onto magnets
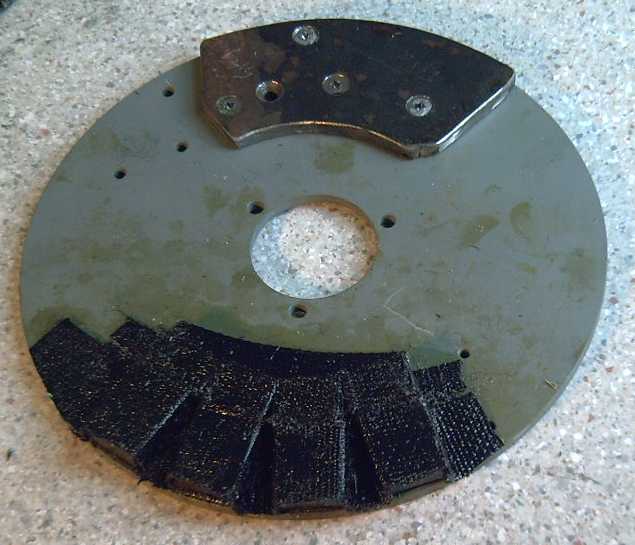
Input/magnet rotor
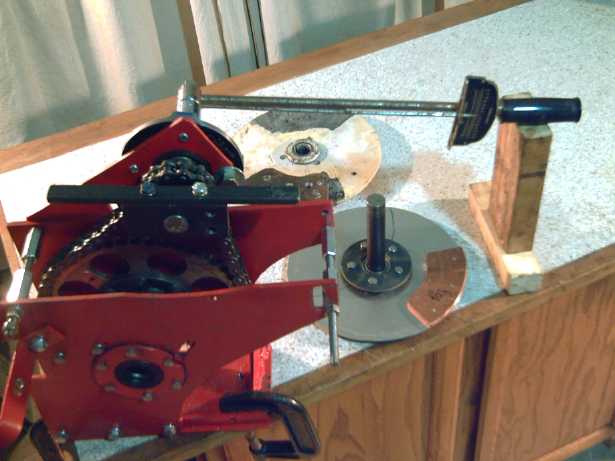
Pieces of the torque converter: 'anvil' arm on output rotor, copper
block side of hammer rotor,
input rotor (before refinishing)
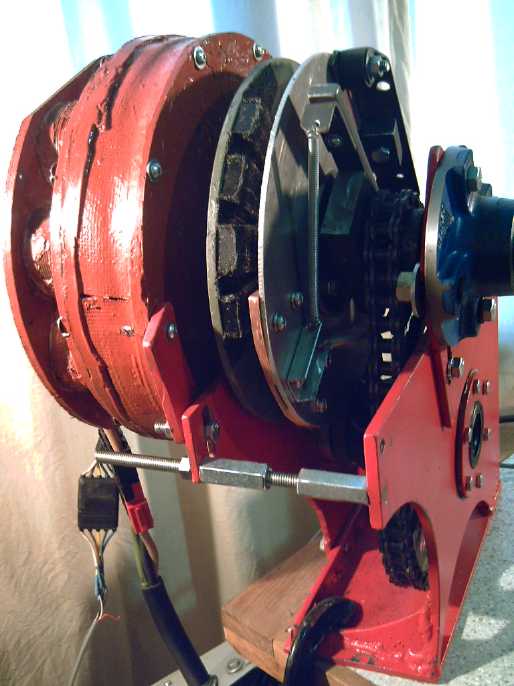
"Finished" V3 converter. Left to right:
- motor
- magnet rotor on motor shaft with wedge of 4 supermagnets
- hammer rotor with copper wedge, hammer assembly with rubber cylinder
'hammers', springs to output shaft assembly
- output shaft assembly with anvil arm, the other end of the springs
- chain drive to differential, then bearing hub for output shaft
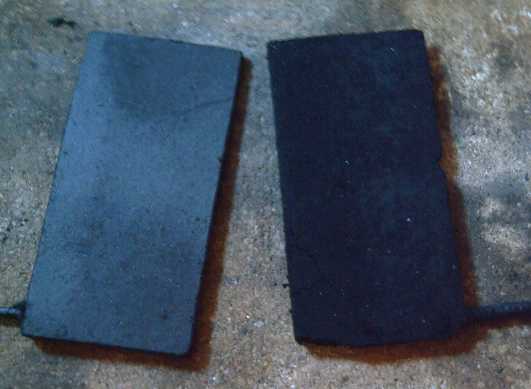
Ni (L) & Mn (R) electrodes as compacted
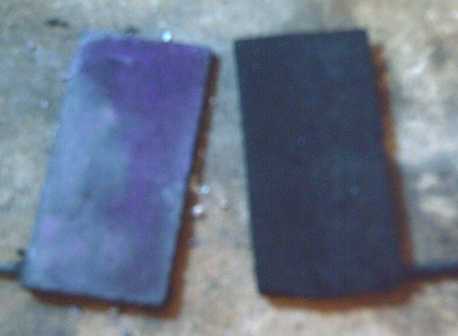
After painting Ni with Ca(OH)2 and torching
The water of painting brought out KMnO4 purple color